Using the OneCellPipe pipeline
Using the OneCellPipe pipeline This is a sub-page of the multi-page documentation for the OneCellPipe pipeline of OneCellBio. The configuration files If you run into trouble or your setup is somewhat special, the…
More tips for running the OneCellPipe
This is a sub-page of the multi-page documentation for the OneCellPipe pipeline of OneCellBio with additional tips.
Analysing inDrop raw data with the OneCellPipe pipeline
Analysing inDrop raw data with the OneCellPipe pipeline In short Described here is a software wrapper around the indrops software for processing single-cell sequencing data generated using 1CellBio's inDrop sequencing technology. The software…
RNA-Seq data quality scores
There are different ways to encode the quality scores in FASTQ files from Next-generation sequencing machines. This is important to find out before using the data and to convert between formats if…
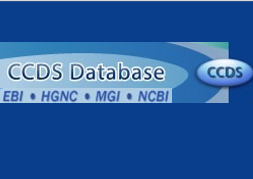
The CCDS project
The CCDS project tries to identify a core set of human and mouse protein coding regions in a stable manner.
- « Previous Page
- 1
- …
- 4
- 5
- 6
- 7
- 8
- …
- 10
- Next Page »